Integrated Photovoltaics: Next-Gen Energy Solutions
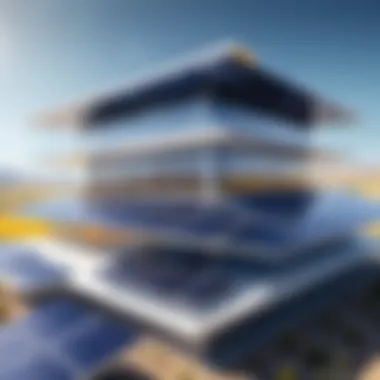
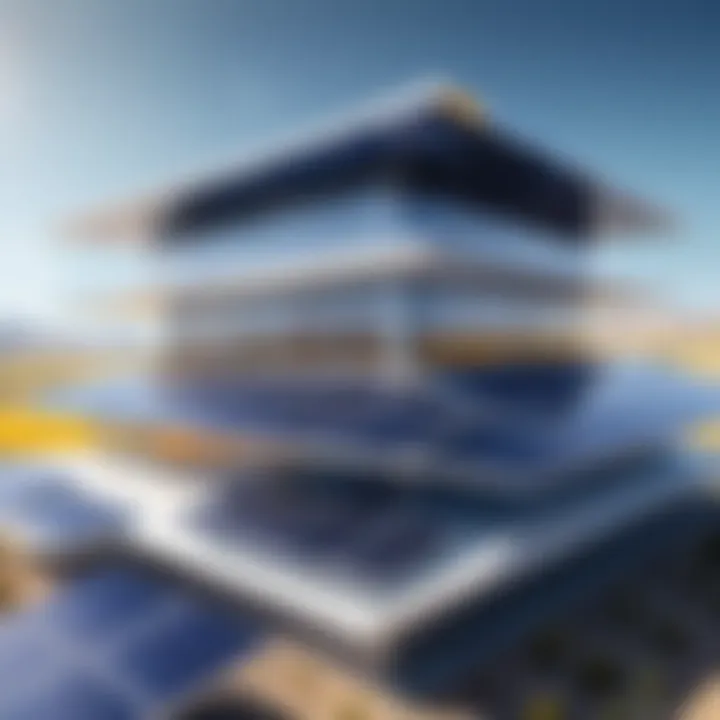
Intro
Integrated photovoltaics represents a pivotal shift in our energy landscape. With the demand for sustainable energy solutions intensifying, these systems offer promising pathways to meet modern requirements for energy efficiency and environmental sustainability. This article examines the principles underlying photovoltaic technology and the methods of integration into architectural designs. We will also explore their applications, economic implications, and case studies that exemplify the transformative potential of these technologies.
Technological Research Overview
As the field of integrated photovoltaics evolves, recent innovations emerge that significantly impact various industries. Understanding these advancements is crucial for decision-makers and stakeholders who aim to leverage energy solutions effectively.
Recent Technological Innovations
Innovations in photovoltaic materials have led to increased efficiency and reduced costs. Companies like SunPower and First Solar are developing bifacial solar panels which can capture sunlight from both sides, thereby enhancing energy output.
Moreover, transparent solar cells are being integrated into window designs. This technology allows buildings to harness solar energy while maintaining aesthetics. The mid-nineties saw thin-film technology gain traction, enabling the integration of solar cells into building facades and roofs.
Impact on Business Operations
The adoption of integrated photovoltaic systems can drive significant operational changes. Businesses can reduce energy costs and enhance sustainability practices, fostering an image of environmental responsibility. This has proven to be a competitive advantage in markets where consumers value green initiatives. Furthermore, many companies report operational savings through efficiency in energy usage, as the reliance on traditional fossil fuels declines.
Future Technological Trends
Looking ahead, we anticipate further advancements in integrated photovoltaics like the use of artificial intelligence to optimize solar panel performance. Machine learning algorithms may predict energy output based on weather patterns, leading to more efficient energy management systems. Also, research is ongoing into energy storage solutions, ensuring that solar energy can be utilized even when sunlight is not available.
"Integrated photovoltaics are not just a technology; they are a commitment to a more sustainable future."
Applications in Building Designs
Integrated photovoltaics can be incorporated into various architectural styles, providing aesthetic and functional benefits. This approach extends beyond traditional rooftop panels, allowing architects to create versatile designs that blend seamlessly with the environment. From solar shingles to facades, the potential applications are vast.
Economic Implications
The economic implications of integrating photovoltaics into buildings are profound. Initial investments tend to discourage some stakeholders. However, long-term savings on energy bills, combined with government incentives, improve the financial viability of these projects.
For instance, incentives like the federal Investment Tax Credit in the United States encourage homeowners and businesses to adopt these systems, leading to a growing market.
Case Studies
Analyzing successful case studies can provide valuable insights into how integrated photovoltaics can make a difference. One noteworthy example is the Bosco Verticale (Vertical Forest) in Milan, Italy, which uses integrated solar panels that contribute to both energy production and ecological purposes. Another case is the Bullitt Center in Seattle, known for its ambitious net-zero energy goal, showcasing how integrated photovoltaic systems can be utilized effectively in commercial real estate.
The End
Foreword to Integrated Photovoltaics
Integrated photovoltaics represent a pivotal advancement in renewable energy technology. Their significance lies not only in harnessing solar power more effectively but also in their ability to blend seamlessly into existing structures and systems. This integration offers a multitude of benefits, ranging from aesthetic enhancements in architectural designs to significant reductions in energy costs. As the world grapples with climate change, the transition towards sustainable energy sources has become increasingly crucial. Integrated photovoltaics can play a vital role in this shift, providing practical and innovative solutions for both residential and commercial settings.
Definition of Integrated Photovoltaics
Integrated photovoltaics, often abbreviated as BIPV (Building-Integrated Photovoltaics), refer to solar energy systems that are incorporated into the design and construction of buildings. Unlike traditional solar panels that are added to rooftops, integrated photovoltaics are integrated into building elements such as windows, facades, and roofs from the outset. This allows them to serve two purposes: generating electricity and fulfilling the role of conventional building materials. As a result, they can help optimize space while also enhancing energy efficiency.
Historical Context and Development
The concept of integrating photovoltaic technology into building materials has evolved significantly since its inception. Early photovoltaic cells were primarily designed for standalone solar panels, limiting their application in urban environments. However, as technology progressed, engineers began exploring ways to marry function with architectural aesthetics. The 1990s marked a notable turning point, with the introduction of innovative materials capable of efficient energy conversion.
During the early 2000s, advancements in both design and efficiency pushed integrated photovoltaics into mainstream architecture. Modern developments have seen an increase in interest from both architects and builders, as the demand for sustainable energy solutions grows. Countries across the globe have started to embrace policies promoting the deployment of integrated photovoltaics. Today, they stand at the forefront of creating energy-efficient buildings that align with sustainable architectural practices.
"Integrated photovoltaics are not just about production; they are about rethinking the way we design and build our living and working environments."
Understanding the historical context of this technology provides insight into its evolution and its potential future applications. As we advance further into the 21st century, integrated photovoltaics are likely to continue developing, leading to more innovative installations and energy optimization strategies.
Understanding Photovoltaic Technology
Understanding photovoltaic technology is vital for comprehending the role of integrated photovoltaics in modern energy systems. The fundamental principles of this technology revolve around converting sunlight into electricity, a process that not only enhances energy efficiency but also aligns with sustainability goals. By grasping how photovoltaic cells operate and the variations in their design, stakeholders can make informed decisions that favor innovation and effectiveness in energy solutions.
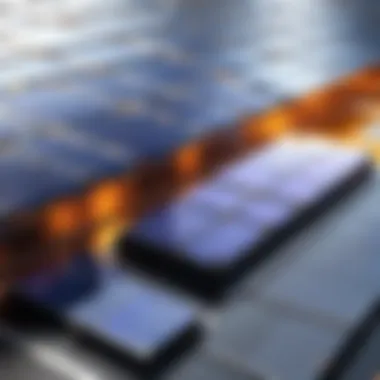
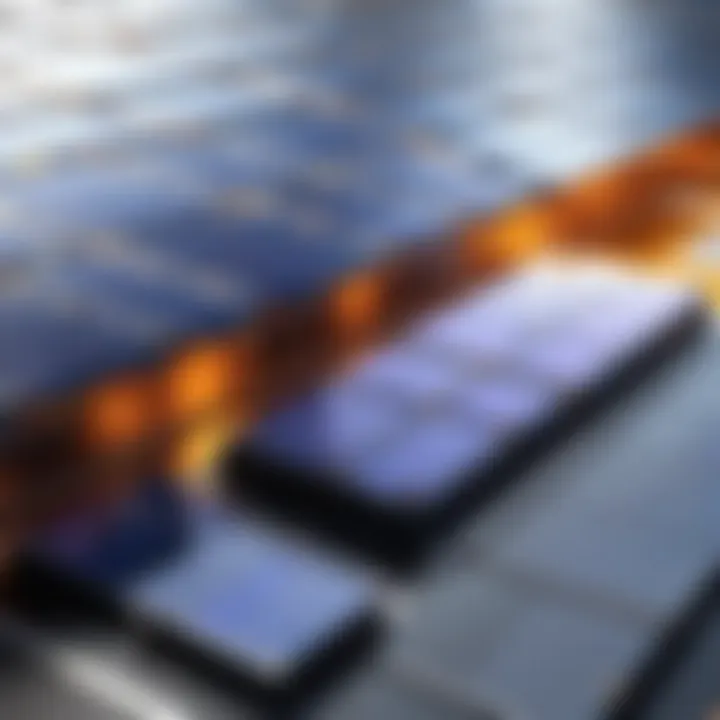
Basic Principles of Photovoltaics
Photovoltaics operate on a simple yet profound mechanism. When sunlight strikes a photovoltaic cell, it generates an electric current through the photovoltaic effect. This effect involves the absorption of photons by semiconductor materials, typically silicon, which excites electrons and generates direct current (DC) electricity. This DC can be converted to alternating current (AC) using inverters, making it usable for a variety of applications.
The process relies on fundamental properties of semiconductors, where impurities are added to create p-type and n-type materials, forming a junction crucial for electric flow. This basic understanding serves as the foundation for advanced innovations in the realm of integrated photovoltaics, where various design and integration methods can maximize energy efficiency and aesthetics.
Types of Photovoltaic Cells
There are distinct types of photovoltaic cells, each with specific characteristics that cater to different applications and preferences.
Monocrystalline Cells
Monocrystalline cells are made from a single crystal structure of silicon. This uniformity results in higher efficiency rates compared to other types, typically ranging from 15% to over 20%. Their key characteristic lies in their space efficiency, meaning they produce more power per square foot. This makes them a beneficial choice for limited space environments, such as urban buildings.
One unique feature of monocrystalline cells is their sleek design, which often appeals to aesthetic considerations in buildings. However, they are more expensive to manufacture, leading to higher installation costs. Therefore, while they offer superior efficiency and longevity, the initial investment can be a drawback.
Polycrystalline Cells
Polycrystalline cells are constructed using multiple silicon crystals melted together. This manufacturing process makes them easier to produce, which generally translates to lower costs compared to monocrystalline cells. The key characteristic of polycrystalline cells is their performance in shaded conditions, where they tend to maintain efficiency better than their monocrystalline counterparts.
A significant advantage is their cost-effectiveness, making them popular for larger installations where space is not as constrained. However, they usually have lower efficiency levels, around 13% to 16%, and may require more extensive space for adequate power output. This trade-off between cost and efficiency plays a crucial role in project decisions.
Thin-Film Technologies
Thin-film technologies involve depositing one or more thin layers of photovoltaic material onto a substrate. These cells are typically lighter and more flexible than traditional silicon cells, allowing for various applications that standard panels cannot satisfy. The key distinct feature is their adaptability to diverse surfaces, including irregular shapes and structures.
Thin-film cells generally have lower efficiency, around 10% to 12%, yet they excel in partial shading situations and high temperatures. Their lower initial cost can also be appealing, especially for large-scale solar farms. However, their shorter lifespan compared to crystalline technologies may necessitate more frequent replacements, influencing long-term economic considerations.
Methods of Integration
The integration of photovoltaic systems into various applications is a cornerstone in advancing the adoption of solar energy. Methodologies for integration provide pathways for harnessing solar power while maintaining or enhancing functionality and aesthetics. This section explains three prominent integration methods: Building-Integrated Photovoltaics (BIPV), Vehicle-Integrated Photovoltaics, and Utility-Scale Integration. Each method has distinct advantages and challenges, and understanding these is vital for industries aiming to leverage solar energy effectively in sustainable practices.
Building-Integrated Photovoltaics (BIPV)
Building-Integrated Photovoltaics epitomizes the union of architecture and energy production. Unlike traditional solar panels that are often seen as external add-ons, BIPV systems become integral to the building's fabric. They can be designed as roofs, facades, or even windows. This integration not only eliminates the visual clutter of conventional solar installations but also contributes to energy savings directly at the site of consumption.
The benefits of BIPV are multifaceted:
- Space Efficiency: By replacing conventional building materials, BIPV systems make efficient use of available space.
- Aesthetic Appeal: They can be seamlessly integrated into aesthetic designs, enhancing the aesthetic value of structures.
- Financial Incentives: Many governments offer incentives for BIPV adoption, which can lead to attractive return on investments.
However, BIPV does present technical challenges. Integration Complexity can become an issue as installation requires skilled labor and adherence to building codes. Moreover, the Performance Variability of BIPV can be influenced by material choices and building orientation. This demands careful planning and design considerations.
Vehicle-Integrated Photovoltaics
Vehicle-Integrated Photovoltaics represents an innovative approach to solar energy application by integrating photovoltaic technology into vehicles. This concept aims to convert unused surface areas, like car roofs and hoods, into energy sources. The primary purpose is to extend the operational range of electric vehicles or to power ancillary systems, such as air conditioning or infotainment devices, thereby increasing overall energy efficiency.
The advantages of this integration method include:
- Extended Range: Solar panels can provide supplementary energy to electric vehicles, mitigating range anxiety for users.
- Energy Independence: Drivers can generate their own power, reducing reliance on external charging stations.
- Low Maintenance: Roof panels typically require less upkeep compared to ground-mounted systems.
However, there are limits to consider. The Capacity Constraints of vehicle roofs limit the total power produced. Additionally, Market Acceptance remains a challenge, as consumers may be hesitant regarding the efficacy of solar technology in vehicles.
Utility-Scale Integration
Utility-scale integration of photovoltaics involves substantial solar installations producing large amounts of energy for grid supply. These systems typically cover vast areas and are crucial in the transition toward renewable energy at an industrial scale. Unlike smaller installations, utility-scale plants benefit from economies of scale, resulting in lower per-watt costs due to bulk manufacturing.
Key benefits of utility-scale integration include:
- High Energy Output: They can produce enough power to support whole cities, providing significant energy contributions.
- Grid Stability: Integrating solar power at a large scale aids in stabilizing energy grids, especially during peak demand periods.
- Centralized Management: Easier monitoring and maintenance than numerous individual installations as operations can be centralized.
Nonetheless, challenges such as Land Use Conflicts arise, as these installations can compete with agricultural land or habitats. Additionally, Regulatory Hurdles must be navigated, as varying regulations can complicate planning and implementation.
"The best integration method depends largely on the specific context and application, each approach needs tailored solutions to achieve optimal performance."
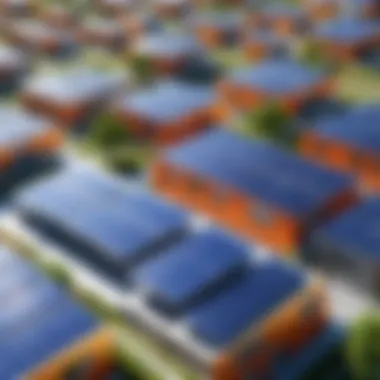
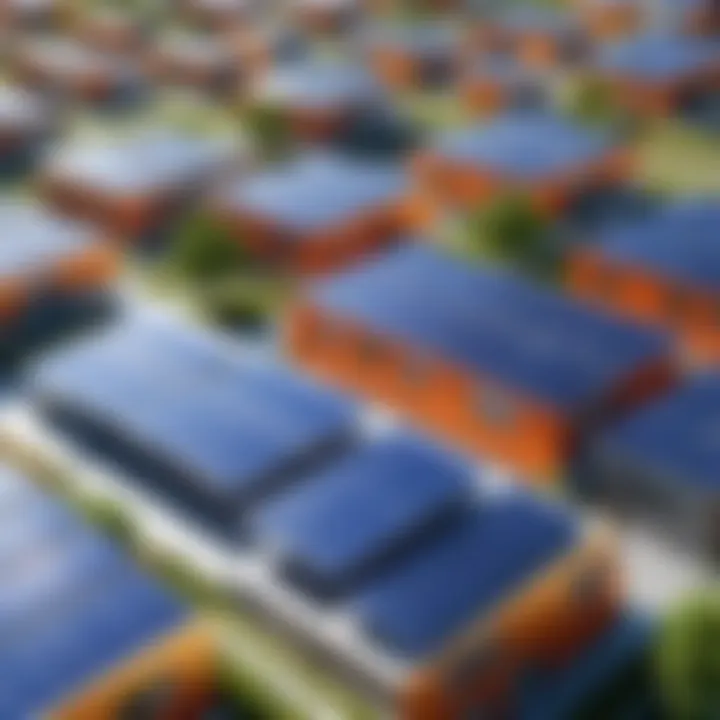
In summary, embracing diverse methods of integration positions integrated photovoltaics as a key player in sustainable energy solutions. Understanding their implications allows stakeholders to make informed decisions, paving the way towards a more solar-centric future.
Applications and Benefits
The role of integrated photovoltaics is significant in shaping the future of energy solutions. By examining their applications and benefits, we gain insights into how these technologies contribute to sustainability, economic growth, and environmental preservation. Integrated photovoltaics not only enhance energy efficiency but also transform infrastructure in ways that benefit society as a whole.
Energy Production and Efficiency
Integrated photovoltaics offer substantial advantages in energy production and efficiency. By embedding solar technology within building structures and vehicles, energy generation becomes an integral part of daily life. This seamless integration allows for harnessing solar energy without the need for bulky panels that compromise aesthetics.
The efficiency of solar cells varies. However, advancements in technology tend to improve their performance continually. Building and vehicle integrated photovoltaics often yield higher energy outputs compared to traditional installations. This is largely due to optimized positioning and exposure to sunlight throughout the day.
Reduction of Carbon Footprint
The potential for reducing carbon footprints with integrated photovoltaics is substantial. By generating clean energy on-site, these systems contribute to lowering greenhouse gas emissions. As buildings and vehicles produce their electricity, reliance on fossil fuels diminishes significantly.
Communities that adopt integrated photovoltaics can reveal meaningful reductions in their overall environmental impact. Governments and organizations often measure these improvements quantitatively. Importantly, this technology aligns with global goals for reducing climate change effects while fostering a greener future.
Economic Advantages
Cost Savings
Cost savings is an essential feature of integrated photovoltaics and their broader adoption. By producing energy on-site, buildings benefit from lower energy bills. This results in positive cash flows over time. While the initial investments may seem high, the long-term reductions in energy costs justify the expenditure.
Moreover, as energy prices rise, the economic appeal of these systems increases. They provide a hedge against future costs. Additionally, excess energy production can generate revenue through sales back to the grid in many regions.
Stimulating Job Growth
Stimulating job growth is another essential aspect of integrated photovoltaics. As demand for solar technologies increases, so does the need for skilled workers. This includes roles in manufacturing, installation, and maintenance.
The solar industry is also expanding into research and development, which encourages innovation and job creation. Communities that embrace integrated photovoltaics can see benefits in their local economies through increased employment opportunities within the renewable energy sector. This approach can help diversify job markets, aiding resilience against economic downturns.
Potential for Government Incentives
Government incentives significantly impact the adoption of integrated photovoltaics. Many countries offer financial assistance, tax credits, and rebates to encourage investments in renewable energy. Such incentives make initial costs more manageable for businesses and homeowners.
In addition, incentive programs can accelerate the transition to sustainable energy practices. They often include provisions for different types of integrated photovoltaics, encapsulating both commercial and residential applications. These government interventions are a critical component in making this technology more accessible and widespread.
"Integrated photovoltaics not only contribute to environmental sustainability but also provide significant economic benefits through innovative job creation, cost savings, and encouraging governmental support."
Challenges and Limitations
Understanding the challenges and limitations associated with integrated photovoltaics is crucial for making informed decisions in energy management. Despite their benefits, integrated photovoltaics come with technical and regulatory hurdles that need addressing. This section examines these obstacles, which can impact the effectiveness and adoption of these technologies.
Technical Challenges
Integration Complexity
Integration complexity is a major aspect of deploying integrated photovoltaics. This issue arises when attempting to merge photovoltaic systems with existing structures or vehicles. The main characteristic of this complexity involves ensuring compatibility between solar technologies and traditional architectures. Such integration can be technically demanding, requiring specialized knowledge in engineering and design.
These challenges make integrated photovoltaics a less popular choice in some situations due to increased project costs and longer timelines. On the other hand, if executed correctly, integrated systems can enhance aesthetic appeal and overall building functionality. A unique feature is the need for customized solutions, which often leads to innovative designs tailored to specific structures. However, this customization can also contribute to variations in installation efficiency, posing disadvantages for quick deployments.
Performance Variability
Performance variability is another key challenge associated with integrated photovoltaics. Variability relates to differences in energy output based on environmental conditions such as sunlight, temperature, and shading. This issue affects the reliability of energy production and can create challenges in energy forecasting. The critical characteristic of performance variability is its unpredictability, which is a significant consideration for project stakeholders.
In terms of benefits, understanding performance variability can lead to improved energy management strategies. For instance, accurate forecasting can help mitigate some of the negative impacts by improving system design and positioning. A unique aspect is that, with ongoing advancements in battery storage technologies, there can be potential solutions to reduce the effects of variability. Nonetheless, these advancements require investment and forward-thinking approaches.
Regulatory and Policy Issues
Regulatory and policy issues play a pivotal role in the adoption of integrated photovoltaics. Governments often set the framework for how solar technologies are integrated into existing energy systems. Challenges arise when regulations are outdated or do not adequately encourage innovation in integrated photovoltaics. Furthermore, inconsistent policies across regions can create barriers for manufacturers and installers, complicating market penetration.
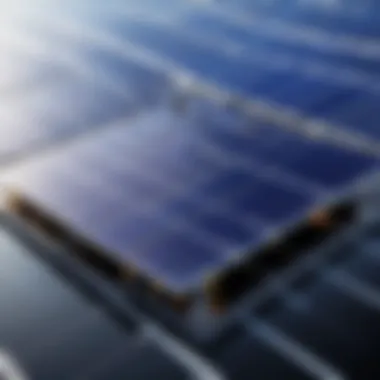
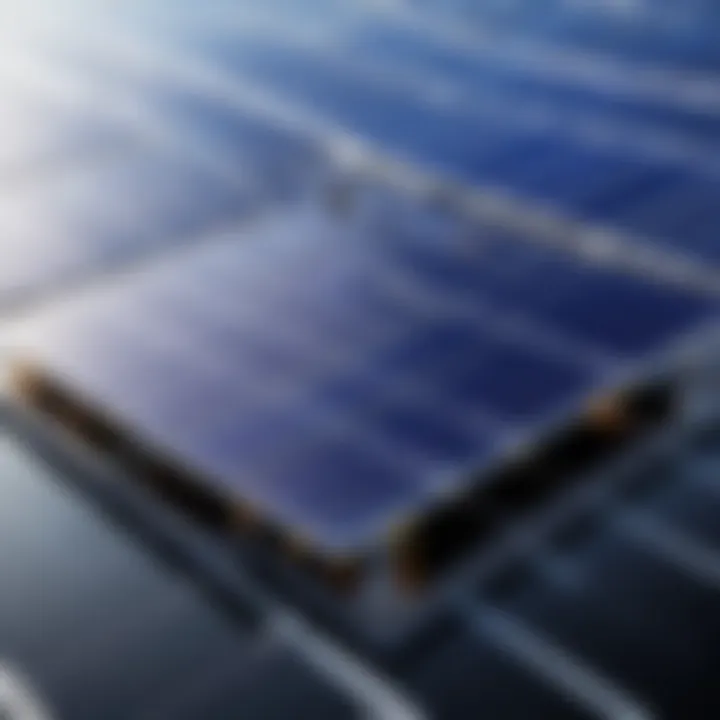
Understanding the regulatory landscape is crucial for businesses in this space. Current incentives, such as tax breaks or grants, can promote the adoption of integrated photovoltaics. However, the lack of clarity in policies can deter investment and complicate project financing. To advance integrated photovoltaics, it will be essential for policymakers to create stable regulatory environments, fostering innovation and investment.
"Navigating the regulatory landscape is crucial for unlocking the full potential of integrated photovoltaics. Consistent and clear policies encourage investment and technological advancement."
Case Studies
Case studies offer critical insight into the real-world application of integrated photovoltaics. They allow stakeholders to examine successes and failures, helping to evaluate the viability of implementations in diverse settings. By showcasing practical examples, these studies illustrate the performance, challenges, and solutions found in actual projects. Moreover, they provide a blueprint for future developments and inspire confidence in potential investors and practitioners.
Case studies present evidence of how integrated photovoltaics can enhance energy independence and sustainability. They reveal the potential economic benefits and operational savings while also drawing attention to the regulatory hurdles that may arise. Readers can better grasp the implications for scalability and market adoption of such clean energy technologies by analyzing the lessons from these projects.
Successful BIPV Projects
Building-Integrated Photovoltaics (BIPV) projects have gained traction globally, exemplifying innovation in architectural design and energy efficiency. One prominent example is the Bullitt Center in Seattle, which is marketed as the greenest commercial building in the world. The building utilizes 570 solar panels, which generate more energy than it uses, making it a model of sustainability. It serves as a potent showcase for how BIPV can transform urban landscapes into energy-efficient environments.
Another significant project is the Bosco Verticale, or Vertical Forest, in Milan, Italy. This residential complex integrates approximately 9,000 plants alongside photovoltaic modules. The design not only enhances energy generation but also supports biodiversity in congested urban areas. Projects like these emphasize the ability of BIPV to harmonize functionality and aesthetics, contributing to the movement toward sustainable architecture.
These successful implementations underline the feasibility and advantages of utilizing BIPV in modern construction, promoting both energy efficiency and architectural beauty.
Innovations in Vehicle Integration
Vehicle-integrated photovoltaics represent a frontier in energy solutions that blend solar technology with transportation. Recent innovations in this sector highlight the potential for electric vehicles to become self-sustaining in their energy needs. A landmark project is the Lightyear One, an electric car with integrated solar cells on its surface. The design harnesses sunlight to extend the vehicle's range, reducing dependency on charging infrastructure.
Moreover, solar panels embedded into vehicles, like those found in certain models of the Toyota Prius, can help power auxiliary systems like air conditioning or infotainment, thereby improving overall energy efficiency.
This innovation period signifies a shift from traditional vehicle designs, as companies explore integration strategies that contribute to greater energy autonomy.
The future development of vehicle-integrated photovoltaics will likely focus on improving efficiency and affordability, ultimately leading to broader adoption and paving the way towards more sustainable transportation solutions.
Future Trends in Integrated Photovoltaics
The evolution of integrated photovoltaics is not static. Understanding future trends in this field is essential for stakeholders aiming to capitalize on upcoming opportunities. These trends are likely to shape the trajectory of energy consumption and sustainability. Staying informed of these developments can provide insights into investment decisions, technological advancements, and market shifts.
Technological Innovations on the Horizon
Technological advancements are pivotal for enhancing integrated photovoltaics. Innovations in material science are leading to the development of more efficient solar cells. The emergence of perovskite solar cells showcases a significant leap in efficiency levels. These cells promise to be cheaper to produce while achieving higher performance metrics compared to traditional silicon cells.
Moreover, the field of transparent photovoltaics is gaining traction. Integrating these into windows would allow buildings to generate electricity without obstructing natural light. Such solutions can transform urban landscapes and enhance energy independence.
In addition to materials, improvements in energy storage technologies are crucial. The synergy between integrated photovoltaics and advanced battery systems can optimize energy use throughout the day. For instance, developments in lithium-sulfur batteries may provide greater energy density. This could make solar energy stored during peak production hours more accessible when demand surges later.
"The rise of smart grids will empower individuals and businesses to manage energy consumption and streamline efficiency."
Shifting Market Dynamics
Market dynamics related to integrated photovoltaics are constantly evolving. As awareness about sustainable energy grows, consumer demand is driving innovation and investment. Governments worldwide are introducing incentives to spur the adoption of these technologies. For example, the U.S. government has implemented various tax credits for residential solar installations, encouraging broader market engagement.
Furthermore, the role of corporations in this landscape is changing. Many businesses are committing to renewable energy targets. This strategic pivot not only reduces their carbon footprint but can also lead to significant long-term cost savings. For example, companies like Apple and Google are investing heavily in solar energy and contributing to projects focused on integrated photovoltaics.
The competitive landscape is also transforming, with new entrants emerging alongside established players. Startups are positioning themselves as innovators in this space, often focusing on specific niches. The increasing collaboration between tech companies and energy firms is another trend to watch. Such partnerships aim to tackle challenges associated with large-scale implementation, making integrated photovoltaics a commercially viable solution.
In summary, understanding the Future Trends in Integrated Photovoltaics provides essential insights for professionals navigating this rapidly changing segment of the energy market.
Culmination
The conclusion of this article underlines the substantial influence of integrated photovoltaics on our present and future energy frameworks. With global demands for sustainable solutions increasing, the integration of photovoltaic systems within buildings, vehicles, and large-scale utilities offers a timely strategy for addressing energy production and environmental considerations.
Summarizing the Impact of Integrated Photovoltaics
Integrated photovoltaics serve as a pivotal junction between architecture, technology, and energy management. Their ability to transform surfaces into energy-generating components not only optimizes real estate but also decouples energy consumption from traditional grid reliance. This aspect is particularly crucial as the world pivots towards decentralized energy production and consumption models.
"Integrated photovoltaics fundamentally alter our approach to energy by embedding production capabilities within the very fabric of our living and working environments."
Key impacts of integrated photovoltaics include:
- Sustainability: By harnessing solar energy effectively, these systems contribute to significant reductions in carbon emissions.
- Economic Viability: Initial investments are often offset by long-term savings on energy bills, alongside potential incentives provided by governments.
- Market Evolution: These technologies are spurring growth in job markets related to engineering, manufacturing, and installation.
Nevertheless, the journey to widespread adoption comes with challenges. Factors like integration complexity and fluctuating performances need careful consideration. Policymakers and industry leaders must collaborate to create supportive frameworks that encourage innovation while addressing regulatory barriers.
In closing, acknowledging the capacity integrated photovoltaics hold for reshaping our energy landscape is critical. Stakeholders across various sectors should recognize their potential not only as energy solutions but also as essential components in the transition towards a more sustainable future.